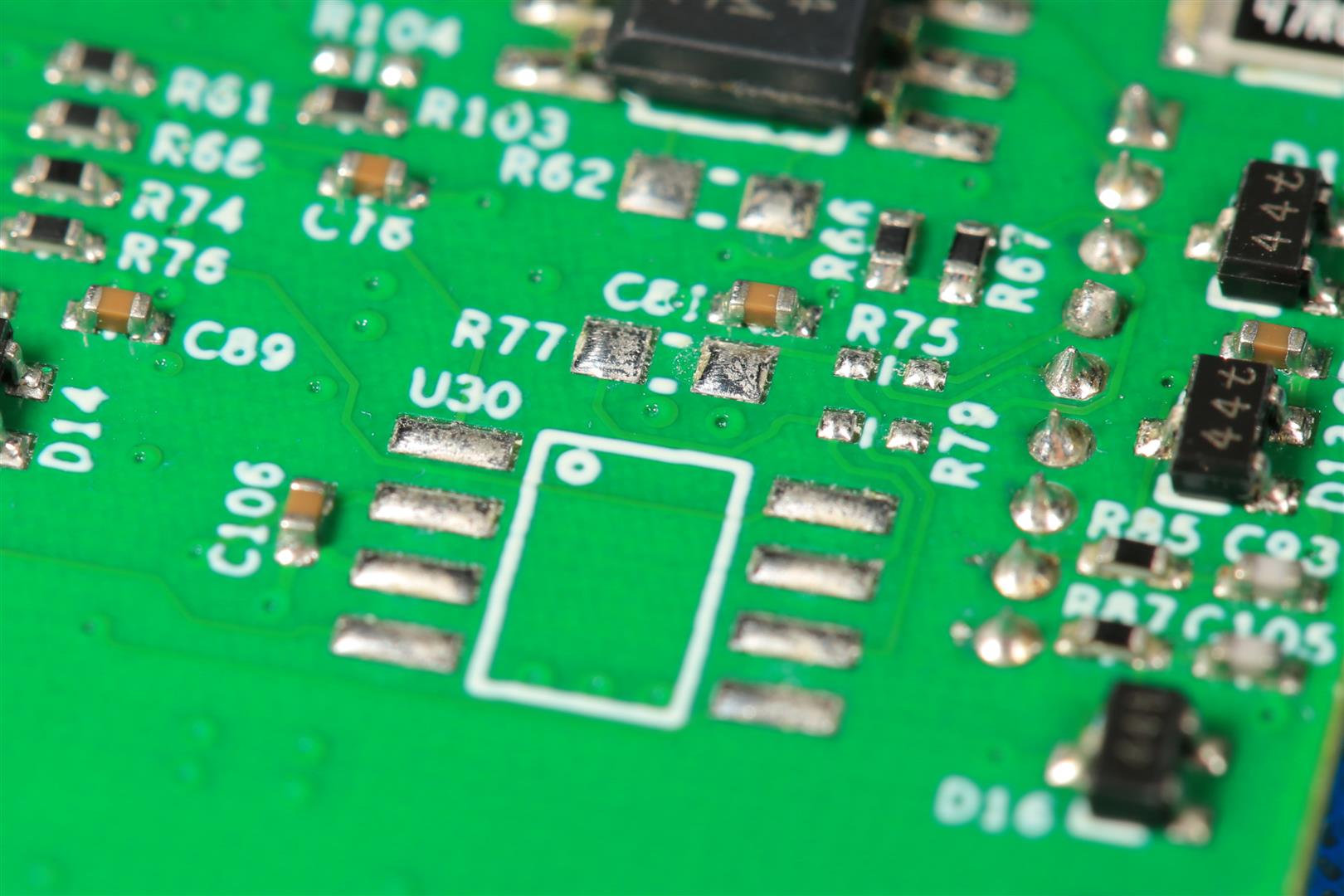
Dealing with the supply crisis in electronics
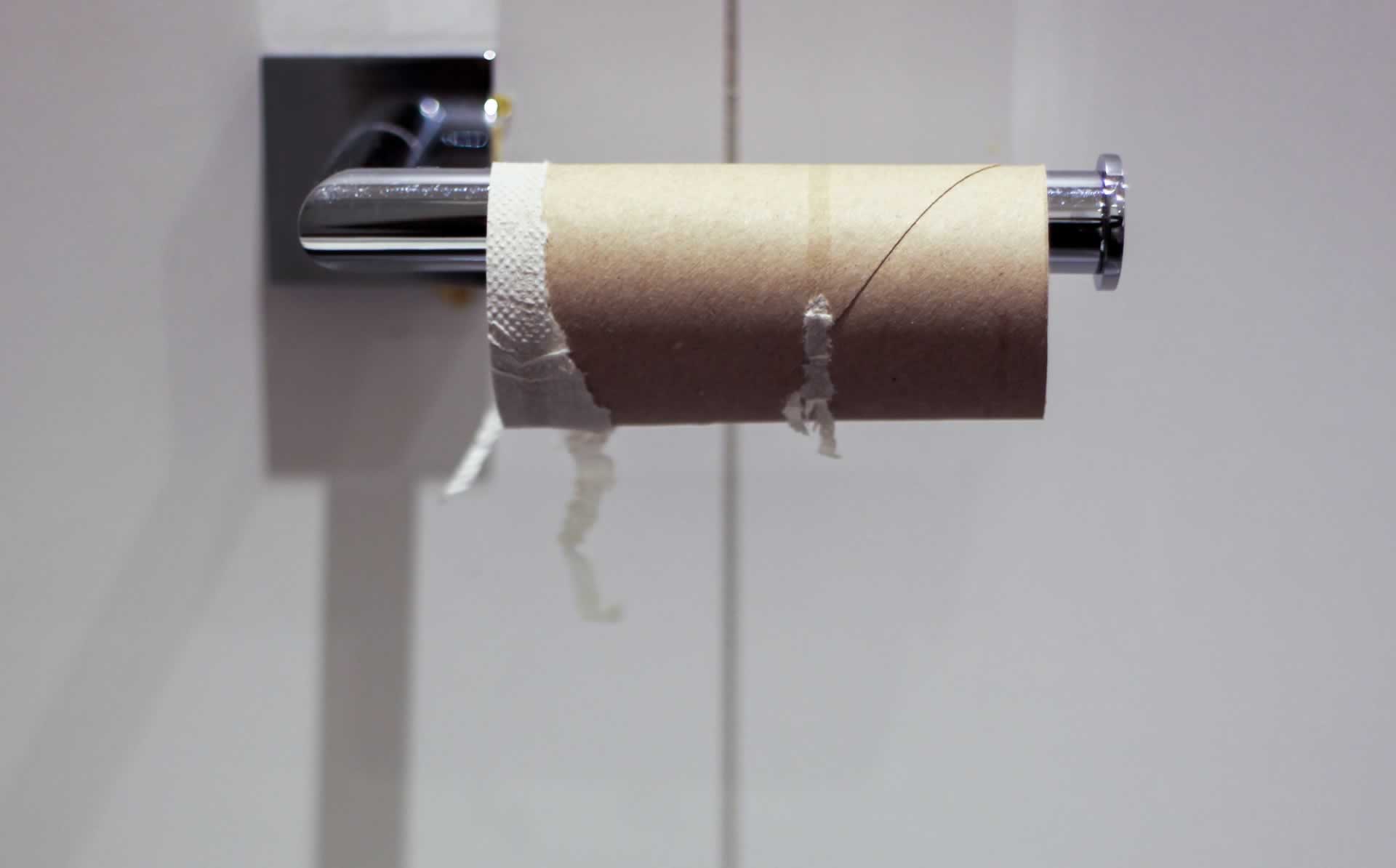
If you’re in the business of making and selling electronic products, you can’t help but have been dismayed by the lack of stock, extended lead times and price hikes which have plagued the supply chain for components over the last year or more.
It doesn’t help that manufacturers are, in many cases, not engaging well with SMBs whose businesses depend on the parts they’ve designed in. Look at the home page for any major IC manufacturer; rather than saying ‘click here for an up to date delivery schedule and to place your order’, it’s still the usual marketing fluff, as though nothing was wrong.
But something is very, very wrong.
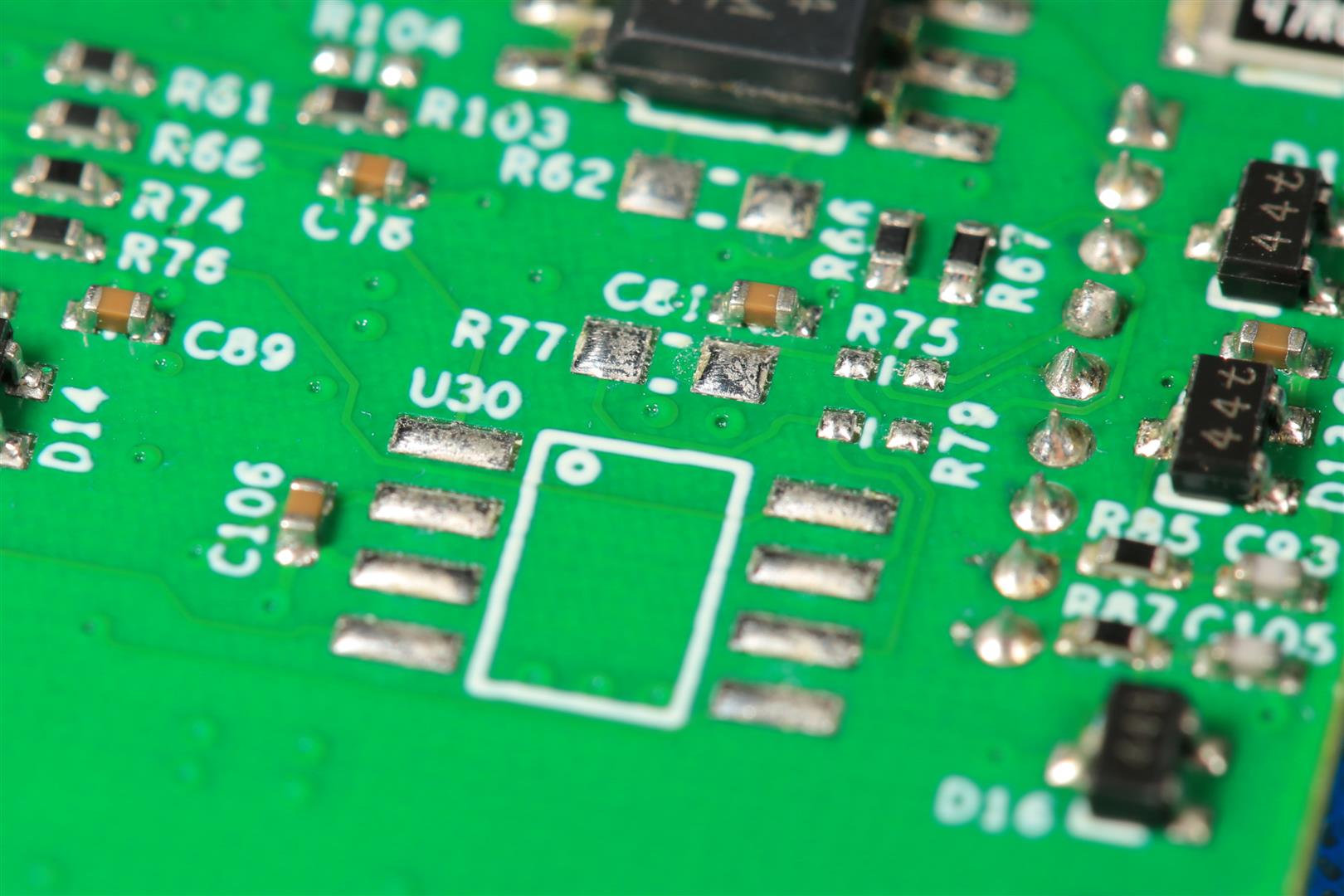
How did we get here?
Various factors have contributed to the shortages:
– Misjudgment of demand by IC manufacturers during Covid lockdown. Instead of sales of IT equipment slowing down as predicted, people instead went out and bought new laptops, cameras and other equipment so they could work from home – a trend which still continues today. Inventory levels throughout the supply chain had been allowed to reduce, only to see a sudden spike in demand.
– Closing of factories in the Far East. As part of their national strategy to reduce the spread of Covid, manufacturing facilities have been closed for weeks at a time, with predictable consequences.
– Hoarding and reselling by speculators. As soon as components arrive with distributors and become available for sale, grey market resellers have been buying them up in bulk, only to immediately try and resell them at an enormous mark-up. Genuine customers, meanwhile, have often had little choice but to pay well over the odds for goods of unknown provenance, or to somehow do without.
– Pestilence, war, famine, and years of chronic [insert practice here] by [insert entity here], depending on who you talk to and their political leanings. Everybody has their own strongly held opinion as to why the market is in quite the state it’s in, most are probably right to some degree or other, and at the end of the day, for us as engineers and manufacturers who just want to stay in business and make a living, it doesn’t really matter anyway.
– Stockpiling by manufacturers. A necessary evil that we’ll get on to…
What can we do?
1) Contact your suppliers
Your first calls should be to the suppliers from whom you purchase components. Just because components aren’t making it to the shelves as free stock doesn’t mean there aren’t any deliveries taking place; components are still being manufactured, but most are being allocated to existing orders since there aren’t enough to go round and clear the backlog at the same time.
If you don’t have an order in place with your distributor (or manufacturer), then you probably won’t be seeing the critical component you need any time soon. If you do, though, then there’s a fair chance that you will – provided you’re prepared to be patient, and possibly pay upfront.
Do be aware, though: delivery dates can and do vary. You may be lucky and get a surprise package from a courier that wasn’t due until next Easter, or you may receive a weirdly unapologetic email telling you your new delivery date is 6 months later than you were promised, with no explanation as to why.
(Spoiler: it’s because the wafer containing your chips was sold to a bigger company with a much larger annual spend on ICs than you have).
2) Buy what you can, when you can
The decision on how many to buy should be straightforward to calculate. Work out the cost of any excess parts you might never use, and compare this with the cost to your business of not being able to manufacture your product because a part is missing.
Buy a number of complete kits – not an excess of any one particular device unless it’s one you use in multiple products – plus spares to cover yield and wastage.
Assume that, when a part becomes available to buy, it might not come up again any time soon.
Bear in mind that it’s far worse to have a 95% complete kit stuck on your shelf, containing thousands of parts you can’t use because something vital is missing, than it is to have a stock of some ‘difficult’ parts that you can at least be sure of using up over time.
A stockpile of complete kits that can be assembled into products is far better for you, and the industry as a whole, than a stockpile of incomplete kits that might sit on the shelf for a year or more.
3) Check… do you need that component at all?
Under normal market conditions, many components are inexpensive and it can make sense to fully populate a board that’s sold in multiple variants, even if some parts on some of those variants will never be used.
The logistical overhead of keeping track of which PCBs have an extra interface, an optional feature, more memory and so on can easily cost more than the parts themselves. You may have always been able to secure a better price by buying a large batch of a fully populated board, than by ordering multiple smaller batches of boards which have parts omitted.
However… right now, some of those parts might be in short supply, so the economics change. Maybe you have a product that comes in 1 channel or 2 channel form, and you only have 100 interface chips to last until next year.
Do you make 100x 1ch boards, or 50x 2ch boards? Both might be good options, and so might any split between the two depending on the orders you have.
Making 50x 1ch boards, each using up 2 of your scarce chips, with the second channel completely unused, is no longer a good idea, though.Review your BoM. Check and see which parts are really needed, and which are left in the kit because it’s usually not economic to omit them. Mark these as DNF. Waste nothing.
4) Check for drop-in alternatives
Most ICs come in multiple variants, and it’s always worth checking the data sheet to see if one variant can act as a drop-in replacement for another.
For example, if you’re making consumer goods, you’re probably designing with the cheapest temperature grade rated at 0 to 70C – but there’s absolutely no reason why you can’t also build your product with an industrial grade part that’ll survive -40 to 85C. Normally you’d choose not to because there’s a small cost difference, but if the industrial grade part is available now and the commercial variant isn’t due to ship for another for 12 months, it’s a virtually zero risk, zero effort change to make.
Some parts are fairly generic and you can switch suppliers. If you’re using general purpose logic, op-amps and similar, then an alternative part with a similar looking part number might be a direct substitute – though it’s always a good idea to check the data sheet spec carefully, and test any previously untried device on a prototype or two.
Sometimes an alternative physical package style is more readily available. If your product uses DIP but you can get the same device in SMT, then a plug-in adapter gets your production line going again. More likely it’s the other way round, but maybe it’s still worth considering how a chip in a different package might be made to fit. Adapter PCBs aren’t that hard to make, if you can’t buy one off the shelf that’s the right type and pinout.
Even if you can’t find an alternative component with a similar part number, it’s often the case that something will fit the bill and work as a functional drop-in replacement, even though its spec is different. Provided the spec of the alternative part is OK in the ways that matter to your design, there’s a good chance it’s usable.
It may even be that you can test and approve a newer device that wasn’t around when your product was developed, and which is functionally superior, or cheaper, than the original.
5) Redesign
If you can’t get hold of a component you need, and there’s no drop-in alternative available either, then it may be time to consider a redesign.
The good news is even in today’s market, that there are components out there available to buy which can perform most functions. You might, for example, not be able to buy a certain voltage regulator, or op-amp, or FPGA, but that doesn’t mean there aren’t functionally similar components that could do the same job. You may be able to buy these off the shelf today, ready to incorporate into your next build with no interruption to your customers and no lost business as a result.
Tip: the very newest ICs, which have yet to be designed in by major OEMs, are often much more readily available than older parts which are snapped up straight away by purchasing departments working to a BoM. Take advantage of the greater speed at which you’re able to work compared to a bigger company with more internal hoops to jump through.
A redesign doesn’t have to be a massive undertaking, particularly if you have the original CAD data for your product. For example, a different voltage regulator probably comes in a different package, and needs a change to the PCB footprint, but that may be the full extent of the changes to your board which are needed. Build a small prototype batch, make sure they work just as before, and you’re back in business – literally.
It’s a bigger job if the part being replaced is special or complex. Changing a CPU or FPGA might be fairly straightforward in hardware terms, but require code to be recompiled, hardware drivers to be rewritten, and thorough testing to ensure everything still works as it should. A uniquely capable analogue component may have no simple replacement available, so that part of your circuit may need more extensive redesign work.
6) Call the experts
If you’re considering that a redesign might help keep production on schedule or your product’s build cost under control, give us a call.
CEL has done numerous redesigns of successful products throughout 2022 for exactly these reasons. Some of these have been very simple, some much more extensive, but all have had one overriding priority: to keep production lines going despite well-known and much loved components suddenly becoming unobtainium.
Our ‘market aware’ design process is along the following lines:
- Review the BoM and identify those components which need to be designed out.
- Review what those components do and the circuits into which they’re incorporated.
- Develop alternative circuits using parts which are known to be available to purchase at the time.
- Purchase the parts for the new design in sufficient quantity to meet foreseeable production requirements.
- When – and only when! – those parts are in the physical possession of the customer, complete the new design and prototype it.
The worst case scenario is that the newly developed board still cannot be manufactured because some component has become unobtainable during the redesign period. Stocking up on each and every line item is the best way to ensure that, regardless of what happens in the market, you’re guaranteed to be able to manufacture a reasonable number of units.
From the sale of these you can recoup the cost of components that you were able to purchase from reputable suppliers at reasonable prices. You’re protected from predatory pricing and other hazards that come with buying on the grey market.
Every new design carries risks, but it’s very unusual to design a high value component into rev ‘A’ of a board, only to design it out again in rev ‘B’.
Most changes between prototypes and production are usually to the wiring (net list), to the values of resistors and capacitors, and the addition of extra parts because it’s always fun to add a new feature at the same time. Unused parts rarely represent a lot of value tied up, and their cost shouldn’t be a major concern, especially compared to the alternative.
What shouldn’t we do?
1) Don’t panic!
The entire industry is going through a difficult time; your customers will understand, and your competitors have it just as bad as you do. We’re all in this together, and that’s unprecedented.
If your prices need to go up to cover your costs, chances are that’s as OK now as it’s ever been. If your lead times need to extend too, that’s also not going to surprise or offend anyone – but if you can keep delivering on schedule while your competitors’ deliveries slip, then you have a competitive advantage that’ll help your reputation no end.
Reviewing your designs and making changes well in advance to avoid delays or significant price hikes can help a lot here.
2) Don’t allow the quality of your product to slip
It might be tempting to waive through a board which is missing a part that’s normally needed for EMC, safety or reliability reasons. Supplies of components like filters and TVS diodes have been among the worst hit thanks to demand from the automotive sector, but they can also be some of the easiest to substitute with alternatives.
Change a footprint, maybe find a way to fit an alternative by hand, but don’t omit something that might lead to a warranty claim or worse. Don’t risk that ‘bad batch’ that harms your reputation long after the market has returned to normal.
3) Don’t buy from suppliers you wouldn’t normally buy from
Most manufacturers have had to at least consider buying components from the grey market over the last year or so. CEL normally advises customers only to ever buy from franchised distributors, but these are strange times and it helps no-one to insist on something that’s just not practical.
Buying from the grey market carries significant risks for the uninitiated, though.
Franchised distributors carry with them the reputation of the manufacturers they represent. They tend to maintain very high standards in terms of component handling and packaging, and follow the correct, necessary precautions against damage from ESD, moisture and mechanical abuse. Components are brand new, genuine, have passed factory testing, and have good shelf life. They usually sell at a fair price too, though under normal market conditions that’s something we all love to debate.
These things are not true of all grey market resellers, though.
Some are, of course, entirely legitimate, reputable organisations who trade genuine, quality product and maintain high standards throughout.
Others are not, and CEL has heard all too many horror stories this year about counterfeit parts, “factory oversupply” chips that turn out to be faulty, and suppliers who think nothing of jacking up the price and/or MOQ of an in-demand component even after an order has been accepted and paid for.
4) Don’t be held to ransom
There are suppliers who advertise good stock of parts which are otherwise hard to find, and chances are the chips simply don’t exist. Or if they do exist, the price is wildly, abusively, coffee-meets-keyboard crazy – and no, you don’t need the chips that badly.
At the very least, haggle hard, and make sure the supplier knows there’s a point at which it makes much more sense to design out their precious chips than be ripped off. Just because they’re asking “double it, then add a zero” compared to the regular price doesn’t mean you need to pay that much, or that anyone else will either.
Ultimately they need to sell the chips that they’ve paid good money for. It’s better for both parties that they sell them (even at a loss) to a genuine OEM who needs them, then not sell them at all. They may need reminding of this, even if it’s not what they want to hear.
5) Don’t go it alone
The current state of the components market is a major challenge for us all.
With over 25 years of design experience to draw upon, CEL has helped customers successfully keep their production lines going throughout 2022, and has the expertise to help you do the same – whether your electronics are a CEL design or not.
Need Help ? Contact Us

Electronics Product Design
Whether you have a detailed specification or just a bright idea, we can help you turn it into a production ready design, backed up by all the CAD data and software, plus the support you need for as long as you need it.

Fix & Improve
Do you have an existing design with a problem, or which doesn’t quite do what you need it to? CEL can help make it better.

The Process
We’re keen on communication. Let us take the time to really understand what you need, and explain how we can help.

Specialist Areas
Need something a little out of the ordinary? We may well have been there before.